Current research directions
Neuronal model – auditory circuits
Binaural hearing is critical for sound localization and scene analysis, and thus for understanding one’s environment. The binaural auditory circuits at the brainstem level compute two critical binaural cues (ITD and ILD). These two cues are required in human to extract one sound from a background of noise, so that we have the ability to hear what we want to hear in daily noisy environment. Intriguingly, neurons in this circuit are abnormally developed in a number of neurological disorders including autism and Alzheimer’s disease. We investigate the formation, dynamics, and pathology of the binaural circuit in animal species that employ similar mechanisms for processing the low frequency range of acoustic signals that covers human speech.
Current Projects
- Explore the nature of auditory dysfunction in fragile X syndrome
- Binaural hearing deficits in fetal alcohol spectrum disorders
- FMRP mechanisms of synaptic establishment and specialization
- Afferent influence and critical period of neural dynamics
1. Explore the nature of auditory dysfunction in fragile X syndrome
Fragile X syndrome (FXS) is the most frequent single gene cause of autism spectrum disorder. It is caused by a loss of the Fmr1 gene product, the fragile X mental retardation protein (FMRP). FMRP is a key regulator of neuronal growth and plasticity, loss of which leads to the characteristic phenotype of FXS, which includes intellectual disability, auditory dysfunction, delayed language development, and difficulties in social communication.
Develop and characterize a gerbil model of FXS. Human use low frequency sounds for vocal communication. Given the central role of sound segregation and vocal communication in language development and social interaction, studying how FMRP loss impairs neuronal processing of low frequency sounds is expected to provide an important and likely necessary avenue for understanding FXS pathology. Current mammalian animal models of FXS are developed in mice and rats, two species that are not sensitive to the low frequency sounds.
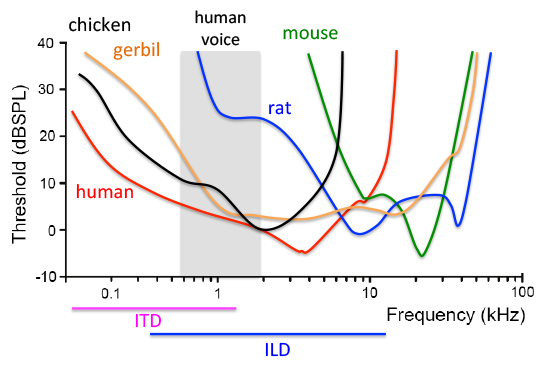
Audiograms of human, rodents and birds. The lower the threshold is, the higher the sensitivity is to a particular frequency of the sound. Hearing is most sensitive for humans at frequencies below 4 kHz and for mice at 16 kHz. The normal human voice range is roughly 100 Hz–2 kHz (shaded area), which is the frequency range that mice barely hear and rats have a high threshold. In addition, humans use low frequency sounds of up to 1.4 kHz for computing interaural time differences, the main binaural cue for hearing with background noise. Gerbils and chickens share an excellent sensitivity to low frequency sounds as humans.
In collaboration with the University of Washington (Curnow), we are developing a loss-of-function model of Fmr1 in the Mongolian gerbil (Meriones unguiculatus). Gerbil is a rodent species extensively used for studying auditory processing given their excellent sensitivity to low frequency sounds. Additionally, gerbils have well-developed social structures that depend on effective communication. Like humans, gerbils establish monogamous breeding pairs and display clear influence of paternal parenting on pup development.
We have completed the de novo sequencing and initial annotation of the gerbil genome (Zorio wt al., 2018). The Whole Genome Shotgun sequence data of gerbil has been deposited at DDBJ/ENA/GenBank. In Fmr1 knockout gerbils, we determine whether low frequency and binaural hearing is compromised in knockout gerbils with auditory functional and behavioral tests, followed by identification of underlying cellular mechanisms of these deficits.
2. Binaural Hearing Deficits in Fetal Alcohol Spectrum Disorders
Difficulty with speech recognition in daily life (e.g., in background noise) has been identified as a common problem in neurodevelopmental disorders (NDs). Speech recognition problems have profound negative consequences for language development, social communication, and intellectual maturation. We aim to understand and correct the pathology of the core auditory circuit that allows auditory perception of speech in noise at the outset. This knowledge will also facilitate for developing therapeutic and intervention approaches for diagnosing and treating NDs.
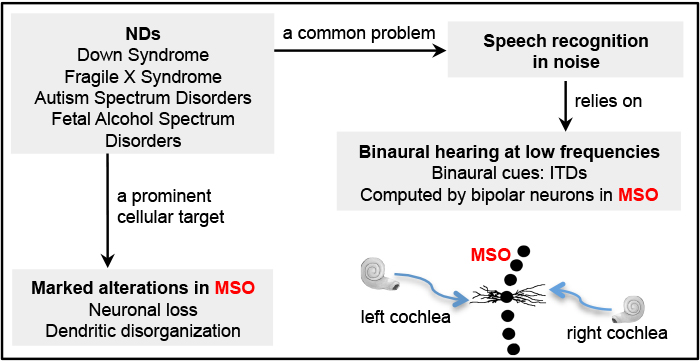
Humans use low frequency sounds for computing interaural time differences (ITDs), a main binaural cue for sound segregation including speech recognition in noise. A key structure for computing ITDs is the medial superior olive (MSO), located in the brainstem. MSO neurons are bipolar, with segregated lateral and medial dendritic domains; they receive segregated excitatory input from the two ears as elicited by low frequency sounds. This anatomical specialization is fundamental to ITD computation because it enables accurate temporal comparison between the two inputs.
Postmortem examinations of human brains have clearly demonstrated that MSO is markedly altered in several NDs. We test the hypothesis that the MSO circuit and its function in binaural processing contribute to the development of language, social, and other cognitive phenotypes of NDs. This project focuses on fetal alcohol spectrum disorders (FASD), a neurodevelopmental disease with demonstrated MSO pathology and auditory dysfunction and problems in speech recognition. We explore the involvement of metabolism in ethanol induced MSO pathology and assess whether nutritional supplements correct the alterations in MSO anatomy and function following prenatal alcohol exposure.
3. FMRP Mechanisms of Synaptic Establishment and Specialization
In this project, we aim to understand the fundamental biology of FMRP regulation in brain development in chickens. Chickens are sensitive to low frequency sounds and have been used extensively in studying neuronal development. Importantly, we have the ability to manipulate specific cell types within a circuit with temporal control. We focus on a large and highly specialized auditory synapse, the Endbulb of Held, the gateway of auditory inputs to the brain.
Synaptic development requires interactions among individual cells that make up the synapse. We aim to understand site-specific and age-specific roles of FMRP in endbulb synaptic development. We assess the effect of manipulating FMRP expression in each cell type (and in pairs) during well-defined stages of endbulb development. We combine neuroanatomical, biochemical, physiological (in collaboration with Dr. Yong Lu at Northeast Ohio Medical University), and bioinformatic approaches to dissect out the cell-autonomous function of FMRP in each cell type and determine their interactive influence on endbulb development.
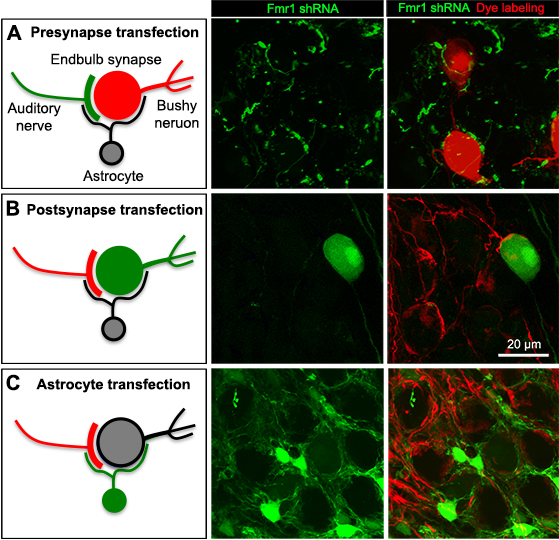
Cell-type specific transfection of the presynaptic axons (cochlear ganglion neurons, A), postsynaptic bushy neurons (B), or local astrocytes (C). Transfected cells/axons are visualized by EGFP (Green), while non-transfected cells and axons are visualized by dye filling, neural tracing or molecular markers (Red). Images were taken from E19 chicken embryos.
4. Shaping Critical Periods of Brain Development
A prominent feature of the brain is its dynamics in response to sensory experience and changes in the environment, the foundation for learning and memory in young brains as well as for brain degradation under pathological or aging conditions. In particular, the brain undergoes significant plasticity from the level of synapses and neural circuits up to behavior, during pregrammed sensitive and critical periods. In neurodevelopmental disorders, notably autistic syndromes including FXS and intellectual disability, children exhibit developmental delays in motor, social and sensory processes and often miss key developmental milestones.
We study formation and regulation of critical periods in brain development, with the ultimate goal of reshaping altered critical periods and restoring normal brain function in neurodevelopmental disorders. Towards this goal, we explore FMRP function in a well-characterized critical period in auditory development in the cochlea nucleus (CN). In mouse models, hearing loss leads to cell death of up to 60% neurons in CN if hearing loss occurs within the first two postnatal weeks. However, it has no affect on cell number if hearing loss occurs just a few days later. We are currently exploring the molecular mechanisms of this critical period and its alteration in FXS.
Major Approaches
Animal models
Chicken embryos - Powerful genetic approaches and easy access to embryonic brains, allowing in-depth investigation of cellular and molecular mechanisms of synaptic and circuit development.
Mouse - Transgenic mouse strains of conventional and Cre-based conditional knockouts; mouse lines that mimic human conditions of hearing loss; AAV-mediated gene-editing with cell group specificity.
Gerbil - An excellent model for studying social interaction and auditory processing due to its similarity to humans.
Protein/RNA analyses: localization, mobility, and interaction: Immunocytochemistry followed by multi-channel confocal microscope imaging allows in vivo determination of protein localization. Protein/RNA mobility and interaction are studied by molecular methods as well as live imaging of genetically labeled molecules.
Sensory functional assays: We test hearing sensitivity and auditory processing ability in animal models under normal and various pathological conditions. Such functional analyses include ABR (auditory brainstem responses), DPOAE (distortion product otoacoustic emissions), acoustic startle, pure-tone exposure, and single-unit extracellular recording.
Microscope imaging: Using laser-based confocal and multi-photon microscopes, we study the structural and biochemical dynamics of brain cells (neurons and glial), as well as their interactions, under control and manipulated conditions. Cell dye-filling followed with 3D reconstruction is combined for single-cell and synapse analyses. Zeiss M2 microscope allows high-resolution montage imaging of large brain areas.
Bioinformatics and Molecular Biochemistry: Targeted gene manipulation is combined with florescent-based cell sorting and quantitative mass spectrometry for identifying differentially expressed proteins under disease conditions (in development). In vitro protein binding and in vivo co-immunoprecipitation studies are conducted to study protein/RNA interaction.
Collaborations
- Electrophysiology recording of auditory neurons: Dr. Yong Lu at Northeast Ohio Medical University
- CRISPR-Cas9 mediated zygote genome editing: Dr. Eliza Curnow at University of Washington
- Alcohol toxicity: Dr. Jennifer Steiner at Florida State University
- Mouse behavior analyses: Dr. Pradeep Bhide at Florida State University
- Protein biochemistry and EV-based brain delivery: Dr. Zucai Suo at Florida State University
Active Grants
NIH R01 MH126176 9/28/2021 – 6/30/2026
Axonal FMRP in synapse development
NIH R56 DC126176 7/1/2023 – 6/30/2025
Axonal FMRP in synapse development
IPRD grant 10/2023 – 10/31/2024
A non-viral treatment for Fragile X syndrome
Completed Grants
FSU Multi-Disciplinary grant 5/1/2022 – 4/30/2023
A novel role of FMRP is prenatal alcohol spectrum disorders
NIH R01 DC013074 1/1/2014 – 12/31/2019
Development and afferent regulation of auditory neurons
FSU PG grant 1/15/2021 – 1/14/2022
A novel role of FMRP in synaptic functionalization by controlling synaptotagmin expression
United States – Israel Binational Science Foundation Research Grant 7/1/2016 – 6/30/2020
Role of Fragile X mental retardation protein in development of brainstem Auditory circuitry
Co-PI with Dr. Dalit Sela-Donenfeld (Hebrew University) and Dr. Edwin Rubel (University of Washington)
NIH R21 DC017267 4/1/2019 – 3/31/2021
Development of a fragile X syndrome model in the gerbil to study auditory dysfunction
Northwestern University Knowles hearing Center Knowles Leadership Fund 12/1/2016 – 11/30/2018
Distinct structure and function of low frequency neurons in the avian cochlear nucleus
Co-PI with Dr. Jason Sanchez (Northwestern University)
Genentech Advanced Neurodegenerative Disease Research 8/1/2016 – 7/31/18
Role of FMRP in brain development
Co-PI with Dr. Diego Zorio (Florida State University)